We develop and apply advanced simulation techniques to predict material behavior over different scales and design materials with improved performances for selected applications. We collaborate with experimental groups to integrate in silico experiments with real experiments for solving problems that are fundamental in nature and yet have a great technological impact.
High-throughput screening of solid interfaces
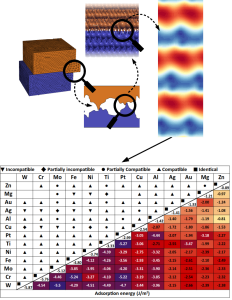
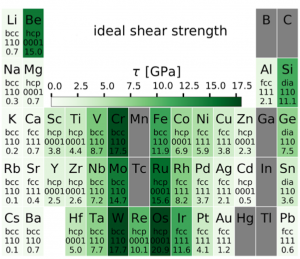
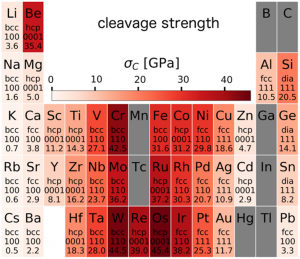
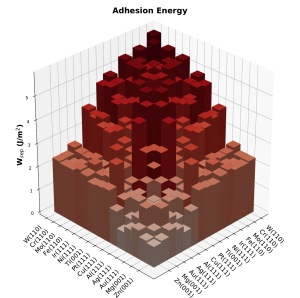
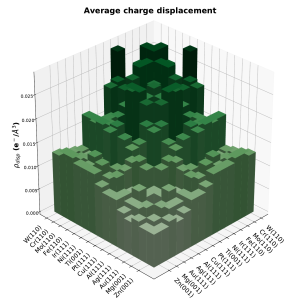
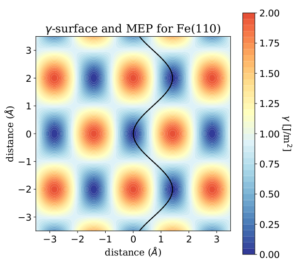
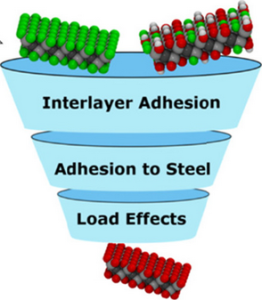
The use of first-principles calculations based on density functional theory (DFT) to screen the properties of thousands of materials represents an exciting opportunity for materials science and applications.
We developed a workflow for the automatized screening of solid surfaces and interfaces. The calculated properties include surface adsorption, interfacial adhesion and charge displacements, ideal shear strength.[47, 53] The latter is obtained from the first-principles calculated potential energy surface (PES) according to the procedure developed by our group [21].
The databases we are continuously populating are available here.
Ab initio and multiscale simulation of system dynamics
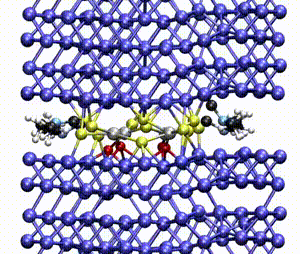
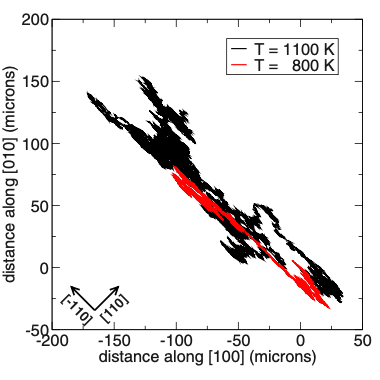
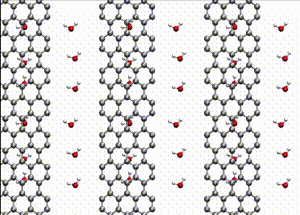
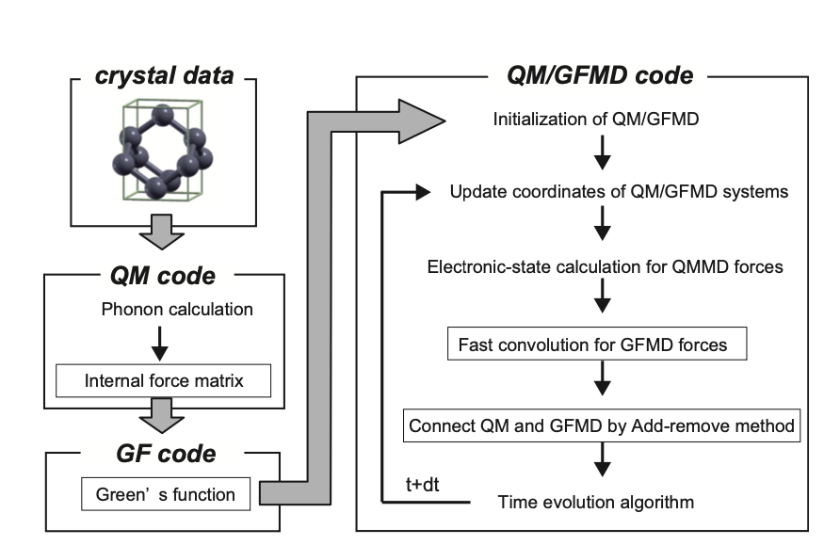
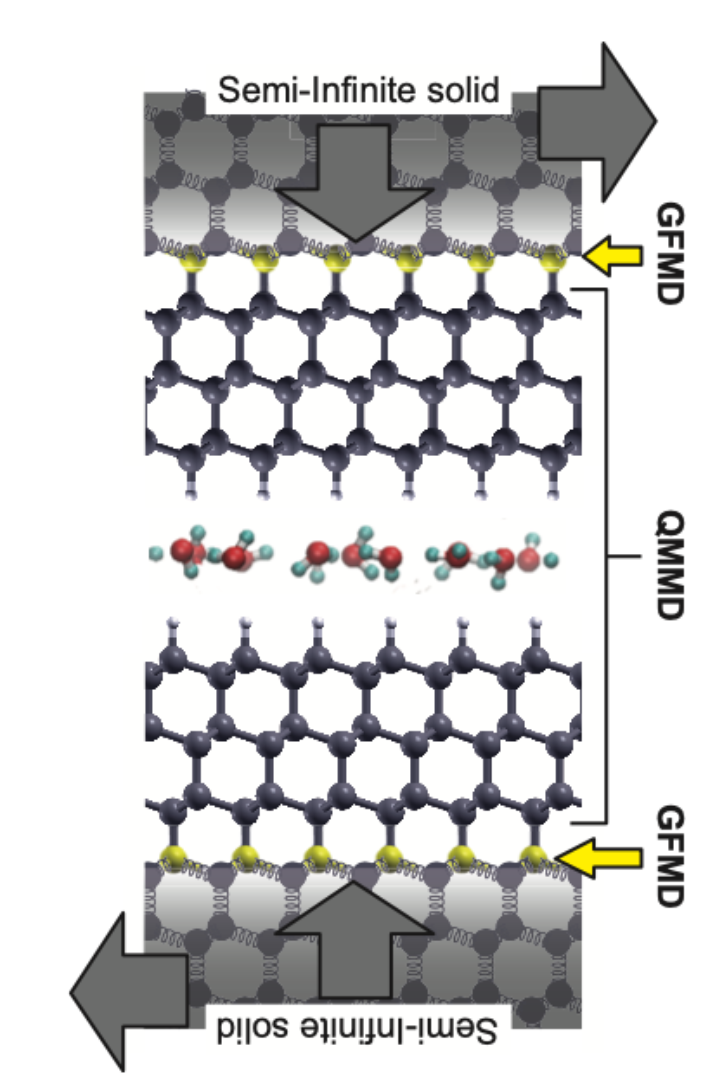
Ab initio molecular dynamics (AIMD) allows for an accurate description of chemical interactions, but its computational cost limits the size and time scale of the simulated system and processes. To overcome these limitations, still preserving the accuracy of AIMD, hybrid schemes can be adopted.
We developed software that links AIMD to Green’s functions molecular dynamics and allows for both the accurate description of interfacial chemistry and the elastic response of a semi-infinite bulk. We have applied it to describe the properties of solid interfaces under load and shear stresses [67].
In the quantum mechanics/molecular mechanics (QM/MM) approach the chemically active region of a system is described by quantum mechanics, while the remaining regions are described by empirical potentials. We successfully applied this approach to describe the chemistry of water molecules at graphene edges [55, 56] and the decomposition of lubricant additives at iron interfaces [58].
Kinetic Monte Carlo (KMC) is a suitable method to describe the evolution of a system when ruled by rare events. We linked first-principles calculations of reaction paths and energy barriers to KMC for simulating surface diffusion and materials growth over macroscopic scales [6, 10, 15].